In this comprehensive 2013 review, C.K. Roberts et al. summarize the mechanisms by which exercise training may affect insulin resistance and thus serve as a tool to either prevent or reverse metabolic syndrome.
As shown in Figure 1 below, insulin sensitivity refers to the body’s ability to dispose of glucose given a fixed insulin load (the x-axis in Figure 1 represents the amount of glucose cleared at a given insulin dose). A variety of evidence has shown that active individuals clear greater glucose with lower insulin secretion than sedentary individuals — that is, active individuals are more insulin sensitive. As sedentary individuals become progressively more insulin resistant, pancreatic beta cells hypertrophy and eventually become unable to secrete sufficient insulin to clear glucose from the blood after a meal. This end state is referred to as glucose intolerance.
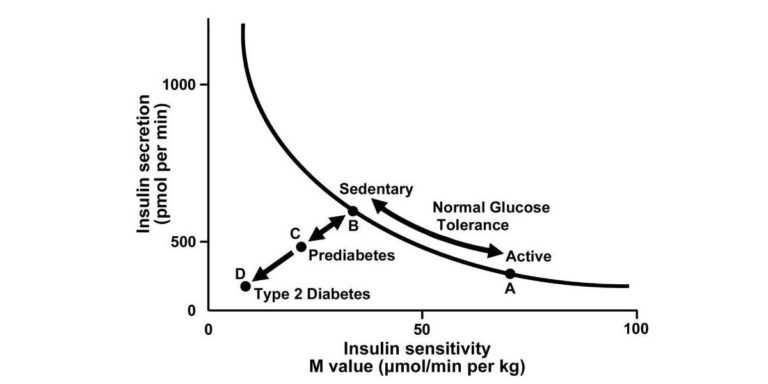
Figure 1: Graph depicting the hyperbolic relation between insulin secretion and insulin sensitivity. Insulin secretion rises as insulin sensitivity falls when an individual goes from a state of exercise training/being physically active (point A) to detraining/sedentary (point B) and vice versa, that is, bidirectionality of the two arrows from B to A when undergoing exercise training/increasing physical activity levels. A failure of insulin secretion to compensate for a fall in insulin sensitivity is noted when both insulin secretion and insulin sensitivity decline from points B to C, leading to elevated fasting glucose and prediabetes (impaired glucose tolerance). A progressive decline in both insulin secretion and insulin sensitivity to point D indicates type 2 diabetes. Adapted from reference (9) with permission.
Trial and observational evidence has shown that aerobic training increases whole-body insulin sensitivity (i.e., the ability of insulin to clear glucose from the blood) and muscular insulin sensitivity (i.e., the amount of glucose taken up by the muscle in response to insulin). Similarly, sedentary individuals exhibit lower whole-body and muscular insulin sensitivity than active individuals. Muscular and whole-body insulin sensitivity are closely connected, as skeletal muscle is the primary tissue insulin stimulates to take up glucose. Thus, the improvements in whole-body insulin sensitivity associated with exercise are largely due to improvements in muscular insulin sensitivity.
The dose of activity has proven important in trials as well; low doses of exercise do not lead to an increase in insulin sensitivity, but the particular threshold for benefit seems to depend on a variety of factors specific to each subject. Similarly, the type of activity plays a role, with various forms of interval training showing consistently positive impacts and resistance training showing benefits independent of any coincident improvements in aerobic capacity. Evidence suggests that aerobic and resistance training have additive effects. As shown in Figure 13, this may be because both types of exercise improve insulin signaling, while only resistance training increases lean body mass (thus affecting the amount of tissue that is able to take up glucose in response to insulin).
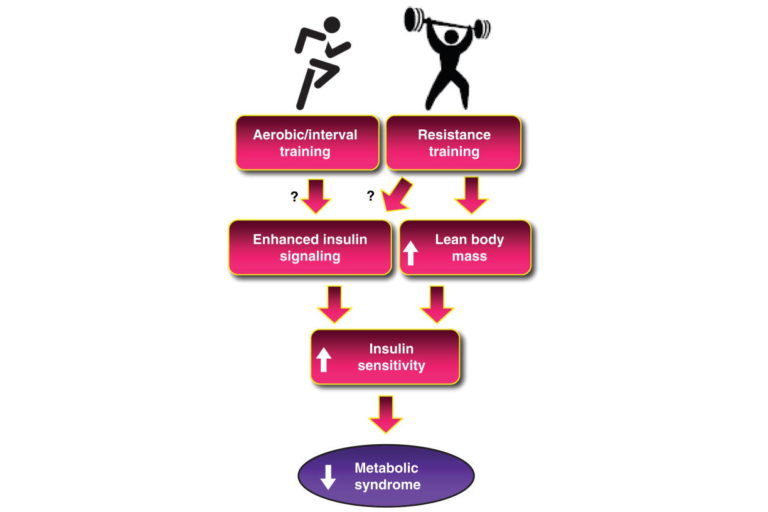
Figure 13: Schematic diagram of future directions to determine mechanisms by which aerobic training (AT) and resistance training (RT) increase insulin sensitivity. Although there is preliminary evidence, more research is needed to clearly identify the mechanisms that are involved, as denoted by the question marks linking AT and RT to enhance insulin signaling.
These effects are particularly prominent in older individuals. Figure 15 shows the dramatic difference in insulin sensitivity (as measured by response to an oral glucose tolerance test, or OGTT) between active and inactive older men; it also demonstrates that this difference is not as pronounced in younger men. Some studies have suggested that the insulin resistance commonly seen in older individuals is not the inevitable result of aging but is due to lower levels of activity. Continuous exercise is required to maintain improved insulin sensitivity, as insulin sensitivity returns to baseline levels after as few as 10 days of inactivity.
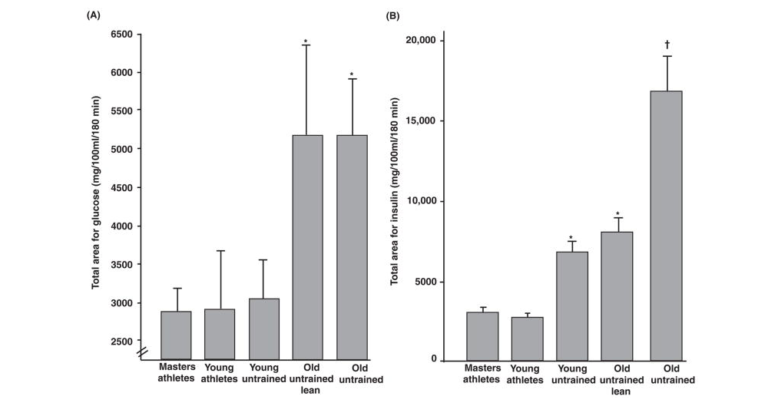
Figure 15: Effects of training and age on area under the curve for (A) glucose and (B) insulin during an oral glucose tolerance test. Adapted, with permission, from reference (577).
In addition to reviewing these findings, Roberts et al. provide an extensive overview of the mechanisms by which insulin sensitivity and/or resistance may develop, some of which have been covered previously on CrossFit.com. One example they discuss is fat accumulation. Figure 5 demonstrates how fat storage in the muscle and liver contributes to insulin resistance in these organs. As the muscle takes on fat, it takes on less glucose in response to the same insulin load, thereby reducing overall insulin sensitivity. As the liver takes on fat, it fails to suppress glucose production in response to an insulin load. In both cases, fat accumulation within the organ has downstream effects that drive chronic elevation of blood glucose levels. Interventions that reduce fat accumulation in these organs — including certain kinds of exercise — may thus improve blood glucose control and insulin sensitivity.
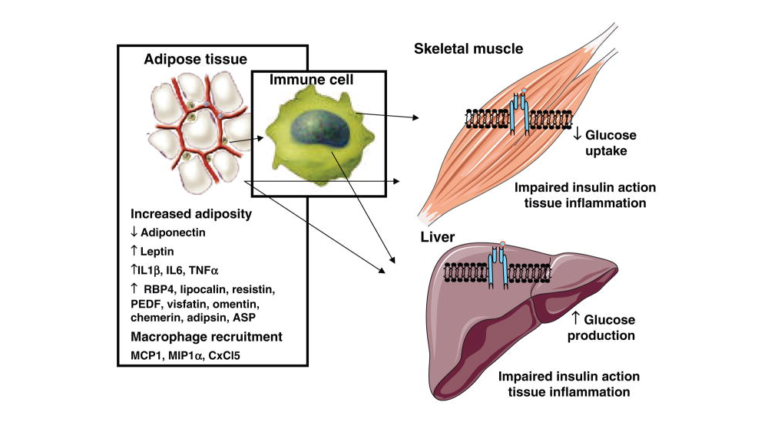
Figure 5: Schematic of adipose tissue-secreted factors that act on muscle and liver to promote insulin resistance.
The paper also discusses the effects of the immune response and a variety of other pathways and regulatory processes. It includes an extensive discussion of the core mechanisms of insulin resistance and the roles different organs, hormones, and signaling mechanisms play in the development of whole-body and organ-specific insulin resistance.
Metabolic Syndrome and Insulin Resistance: Underlying Causes and Modification By Exercise Training