Question: Does intermittent fasting lead to significant changes in young, healthy adults?
Takeaway: This preliminary study indicates 14-hour daily fasts, without a change in dietary composition, change a variety of pathways that may reduce cancer risk and improve metabolic health.
Circadian rhythms are moderated by two “clocks.” The first clock is a “master clock” that responds to daily dark-light cycles, is regulated in the hypothalamus, and regulates various circadian processes in the rest of the body via neuronal and hormonal signals (1). The second clock responds to meal times, and in particular to regular patterns of feeding and fasting (2). This second clock can override the impact of the master clock on organs and tissues if mealtimes and the duration between meals are deliberately manipulated (3).
Disruptions to circadian rhythm have been linked to impaired glucose and lipid metabolism, cancer, the metabolic syndrome, and immune system dysfunction; restoration of circadian rhythm has been linked to the prevention of these same conditions (4). Studies in which mice have been fasted overnight (5) have triggered improvements in a variety of metabolic pathways (6). This April 2020 trial built upon this preliminary evidence by surveying the effect of fasting on a variety of proteins and other biomarkers in young, healthy adults.
Fourteen subjects (13 men and 1 woman) free of chronic disease were recruited to follow a 30-day fasting program. Each day, fasting began after a pre-dawn breakfast and continued until a post-dusk dinner. In practice, this led to a daily fast exceeding 14 hours each day. Subjects ate their usual diets during non-fasting hours and were instructed to not reduce their total calorie intake. Proteomics were assessed prior to, immediately after, and one week after the fasting period.
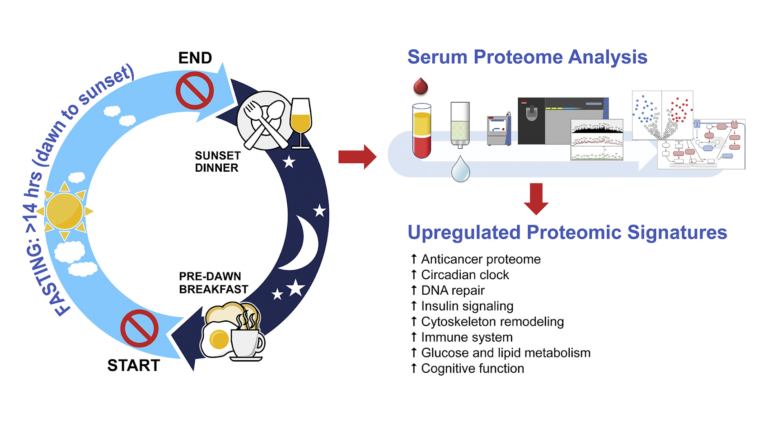
Figure 1: An overview of the intervention
Fasting changed expression of a variety of proteins. Significantly, these changes included:
- Increased expression of LATS1, CFHR1, and COLEC10, all of which are downregulated in hepatic (liver) cancers (7);
- Reduced expression of RRBP1 and FM05, which are increased in colorectal cancers (8);
- Reduced expression of a variety of other proteins linked to increased cancer risk, including B4GALT1, ASAP1, TNSK2, HUWE1, ARHGEG28, PALB2, SMOC1, IRAk, and MUC20 (9);
- Increased expression of CEP164, which regulates DNA repair in response to UV damage (10);
- Changes to HOMER1, APP GP, and ARPP21 GP, indicating reduced risk of Alzheimer’s disease and neurodegenerative disease (11);
- Changes to TMP3, TMP4, PLINK G4, CFL1, and PKM, indicating decreased risk of insulin resistance and the metabolic syndrome (12).
There were no significant changes to traditional biomarkers such as weight or insulin resistance, which may be explained by the fact that subjects were healthy at baseline.
Overall, the researchers concluded intermittent fasting leads to changes in the proteome that are protective against cancer, inflammatory and immune disease, obesity, diabetes, the metabolic syndrome, and various pathological forms of cognitive dysfunction — even when applied to young, healthy humans. Proteomic studies such as this one are at best hypothesis-generating, but this study suggests fasting leads to significant shifts in protein expression that may reduce risk of disease, even in already healthy subjects who are not asked to change the composition of their overall diet. This suggests the use of fasting as a tool to improve metabolic health and lower disease risk, either independent of or alongside changes in overall diet, deserves further investigation.
Notes
- Restricted feeding uncouples circadian oscillators in peripheral tissues from the central pacemaker in the suprachiasmatic nucleus; Collective timekeeping among cells of the master circadian clock; Restricted feeding entrains liver clock without participation of the suprachiasmatic nucleus; Coordination of circadian timing in mammals; Time-restricted feeding entrains daily rhythms of energy metabolism in mice; Entrainment of the circadian clock in the liver by feeding; Resetting central and peripheral circadian oscillators in transgenic rats; The mammalian circadian timing system: organization and coordination of central and peripheral clocks
- Ibid.
- Impact of time-restricted feeding and dawn-to-sunset fasting on circadian rhythm, obesity, metabolic syndrome, and nonalcoholic fatty liver disease
- Circadian rhythms and tumor growth; Role of the circadian clock in the metabolic syndrome and nonalcoholic fatty liver disease; Time-restricted feeding without reducing caloric intake prevents metabolic diseases in mice fed a high-fat diet; Cancer inhibition through circadian reprogramming of tumor transcriptome with meal timing
- Note: Mice are nocturnal eaters, so a nighttime fast for mice is equivalent of a daytime fast for humans.
- Long-term restricted feeding alters circadian expression and reduces the level of inflammatory and disease markers
- Decreased expression of COLEC10 predicts poor overall survival in patients with hepatocellular carcinoma; Identification of 1,4-galactosyltransferase I as a target gene of HBx-induced cell cycle progression of hepatoma cell; Elevated β1,4-galactosyltransferase I in highly metastatic human lung cancer cells: Identification of E1AF as important transcription activator; Estrogen induced β-1,4-galactosyltransferase 1 expression regulates proliferation of human breast cancer MCF-7 cells; B4GALT1 gene knockdown inhibits the hedgehog pathway and reverses multidrug resistance in the human leukemia K562/adriamycin-resistant cell line
- Overexpression of flavin-containing monooxygenase 5 predicts poor prognosis in patients with colorectal cancer; Endoplasmic reticulum ribosome-binding protein 1, RRBP1, promotes progression of colorectal cancer and predicts an unfavourable prognosis; RRBP1 overexpression is associated with progression and prognosis in endometrial endometrioid adenocarcinoma
- Cloning and characterization of TNKL, a member of tankyrase gene family; Immunohistochemical detection of tankyrase 2 in human breast tumors and normal renal tissue; HUWE1 controls the development of non-small cell lung cancer through down-regulation of p53; Alterations of ubiquitin ligases in human cancer and their association with the natural history of the tumor; Gastrin-stimulated Gα13 activation of Rgnef protein (ArhGEF28) in DLD-1 colon carcinoma cells, etc.
- Cep164 is a mediator protein required for the maintenance of genomic stability through modulation of MDC1, RPA, and CHK1; UV-dependent interaction between Cep164 and XPA mediates localization of Cep164 at sites of DNA damage and UV sensitivity
- Homer1a protein expression in schizophrenia, bipolar disorder, and major depression; Scaffold protein Homer 1: implications for neurological diseases; Behavioral and neurochemical phenotyping of Homer1 mutant mice: possible relevance to schizophrenia; Selectively reduced expression of synaptic plasticity-related genes in amyloid precursor protein + presenilin-1 transgenic mice; Successful cognitive aging in rats: a role for mGluR5 glutamate receptors, Homer 1 proteins and downstream signaling pathways, etc.
- An actin filament population defined by the tropomyosin Tpm3.1 regulates glucose uptake; Tropomodulin3 is a novel Akt2 effector regulating insulin-stimulated GLUT4 exocytosis through cortical actin remodeling; The role of cytoskeleton in glucose regulation; Adipose tissue expression of the lipid droplet-associating proteins S3-12 and perilipin is controlled by peroxisome proliferator-activated receptor-gamma; Peroxisome proliferator-activated receptor γ and adipose tissue — Understanding obesity-related changes in regulation of lipid and glucose metabolism; Arp2/3- and cofilin-coordinated actin dynamics is required for insulin-mediated GLUT4 translocation to the surface of muscle cells; Pyruvate kinase M2 activation may protect against the progression of diabetic glomerular pathology and mitochondrial dysfunction
Intermittent Fasting From Dawn to Sunset for 30 Consecutive Days Is Associated With Anticancer Proteomic Signature